ECoG – electrocorticography
Early applications
Learning Objectives
- Be able to describe what electrocorticography is
- Be able to explain why it is done
Electrocorticogram (ECoG) refers to the signal obtained from macroelectrodes (typically 2–3 mm in diameter) placed directly on the pial surface of cortex of epileptic patients for localization of the seizure focus. This recording technique combines adequate temporal and spatial resolution with the lower risks of medical complications compared to the other invasive methods. ECoG is sometimes also known as intracranial electroencephalography (iEEG) and uses many of the same mechanisms as EEG but is an invasive technique reserved for patients who need it.
Since the second half of the twentieth century, (iEEG), including both ECoG and stereo-electroencephalography (sEEG), has provided an intimate view into the human brain. At the interface between fundamental research and the clinic, iEEG provides both high temporal resolution and high spatial specificity but comes with constraints, such as the individual’s tailored sparsity of electrode sampling. Over the years, researchers in neuroscience developed their practices to make the most of the iEEG approach.
In clinical applications, ECoG electrodes are typically arranged into rectangular grids (for example, 6 × 8) or strips containing several electrodes in a single row. Platinum-iridium electrodes are often used, with the diameter of 4 mm most common for clinical applications.
To accurately estimate the epileptogenic cortex or to make inferences about cognitive processes, it is important to determine the “spatial spread” of ECoG (i.e., the extent of cortical tissue that contributes to its activity). Spatial spread is best studied for multiunit activity (MUA) and local field potential (LFP) recorded using microelectrodes implanted in sensory areas, especially in visual or auditory cortices that contain topographic maps, by presenting small visual stimuli or tone pulses.
According to an experiment conducted by Agrita Dubey and Supratim Ray, ECoG spreads were local and not much larger than the size of the macroelectrode. They conducted this research in V1 (a part of the visual cortex) in monkeys and believe that their findings possibly extend to other brain regions as well.
ECoG has the potential to be used in a wide variety of neuroscience research in the coming years to continue exploring neurological disorders and other topics of interest.
Before the first application of EEG in human recordings by Hans Berger in 1929, there was no way to quantify ongoing neuronal activity to help understand normal and pathologic functional states. By 1934, EEG had helped characterize and differentiate normal human brain waves and seizure patterns in patients with brain tumors and epilepsy. By measuring the local voltage fluctuations, clinicians and neuroscientists could identify physiologic and pathologic neuronal activity in superficial regions of the brain. EEGs are recorded from electrodes that are affixed to the scalp. Unfortunately, one flaw in these scalp EEGs is that they are limited in spatial resolution, as a result of recording signals through the skull and intermediate tissue. This is where the advent of ECoG recordings changed how we measure brain waves and seizure patterns.
In conjunction with clinical features, clinicians can diagnose epilepsy and classify patients based on specific epilepsy subtypes, using diagnostic tools such as electroencephalography (EEG), video electroencephalogram (VEEG), computed tomography (CT), and/or magnetic resonance imaging (MRI).
In 1934, the first use of intraoperative ECoG data by Foerester and Altenburger provided improved spatial resolution necessary to quantify electrical activity in both superficial and deep brain structures. Subdural electrode grids and strips were used for direct recording from the cortex, while depth electrodes allowed for recording from deep structures. Analysis of ECoG data became a vital step in clinical decision-making for surgical resection or neuromodulation, and is the mainstay of the surgical epilepsy workup
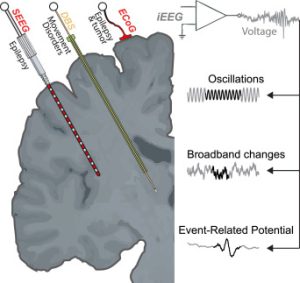
Research Applications of Electrocorticography
Learning Objectives
- Be able to explain at least one way ECoG is used to treat neurological disorders (i.e., clinical applications beyond monitoring and mapping brain function in the hospital)
- Be able to name at least one function of ECoG for basic (non-clinical) neuroscience research
ECoG is routinely used in clinical practice for preoperative cortical mapping in epileptic patients. During the last two decades, research utilizing ECoG has considerably grown, including the paradigms where behaviorally relevant information is extracted from ECoG activity with decoding algorithms of different complexity. Several research groups have advanced toward the development of assistive devices driven by brain-computer interfaces (BCIs) that decode motor commands from multichannel ECoG recordings.
Brain signal decoding promises significant advances in the development of clinical brain computer interfaces (BCIs). In Parkinson’s disease (PD), the first bidirectional BCI implants for adaptive deep brain stimulation (DBS) are now available. Brain signal decoding can extend the clinical utility of adaptive DBS but the impact of neural source, computational methods, and PD pathophysiology on decoding performance are not well known, so one group developed an invasive brain-signal decoding approach based on intraoperative sensorimotor ECoG and subthalamic LFP to predict grip-force (a representative movement decoding application) in patients undergoing DBS. ECoG was found to be superior to other methods for this and provides a neurophysiological framework for invasive brain signal decoding to aid the development of an individualized precision-medicine approach to intelligent adaptive DBS.
Meniere’s Disease, a disease characterized by a buildup of fluid in the inner ear, is sometimes diagnosed and monitored using ECoG. Rather than a doctor simply evaluating a patient’s observations of the symptoms, ECoG provides a more accurate way to diagnose the disease through identification of endolymphatic hydrops that are part of the disease. However, there is some discussion as to whether the addition of invasive technology is needed to accurately diagnose the disease.
Recently, ECoG has also been used in ground-breaking research related to interpreting imagined/internal speech. These advances would allow technology to be able to interpret and display what non-verbal individuals are thinking in a more natural and intuitive way than current practices.
With the recent advances in ECoG technology, there is a ton of promise for this to be a widely-used technique in BCI and other research in the coming years
Attributions
Section 1.
Frontiers | Decoding Movement From Electrocorticographic Activity: A Review (frontiersin.org)
Cortical Electrocorticogram (ECoG) Is a Local Signal | Journal of Neuroscience (jneurosci.org)
Biomedicines | Free Full-Text | Responsive Neurostimulation for Seizure Control: Current Status and Future Directions (mdpi.com)
Section 2.
Electrocorticography is superior to subthalamic local field potentials for movement decoding in Parkinson’s disease | eLife (elifesciences.org)
Biosensors | Free Full-Text | Slippery Epidural ECoG Electrode for High-Performance Neural Recording and Interface (mdpi.com)
Advances in human intracranial electroencephalography research, guidelines and good practices – ScienceDirect
References
Nguyen LT, Harris JP, Nguyen QT. Clinical utility of electrocochleography in the diagnosis and management of Ménière’s disease: AOS and ANS membership survey data. Otol Neurotol. 2010 Apr;31(3):455-9. doi: 10.1097/MAO.0b013e3181d2779c. PMID: 20104195; PMCID: PMC3037765.
Martin S, Iturrate I, Millán JDR, Knight RT, Pasley BN. Decoding Inner Speech Using Electrocorticography: Progress and Challenges Toward a Speech Prosthesis. Front Neurosci. 2018 Jun 21;12:422. doi: 10.3389/fnins.2018.00422. PMID: 29977189; PMCID: PMC6021529.