MEG – Magnetoencephalography
Physical and Physiological Basis
Learning Objectives
Type your learning objectives here.
- Describe what signals magnetoencephalography (MEG) detects.
- Summarize the technology involved in MEG
Magnetoencephalography (MEG) is another technique for noninvasively measuring neural activity. The flow of electrical charge (the current) associated with neural activity produces very weak magnetic fields that can be detected by sensors placed near the participant’s scalp. The number of sensors used varies from a few to several hundred. Like electroencephalography (EEG), the source of the magnetic fields is dendritic current of pyramidal neurons that fire synchronously and in parallel. Axonal and synaptic currents and their magnetic fields cancel out.
MEG is the measurement of the magnetic field generated by the electrical activity of neurons. It is usually combined with a magnetic resonance imaging to get what is called magnetic source imaging. The technology that has helped record these minute magnetic fields is super-conducting quantum interference detector which is like a highly sensitive magnetic field meter. To maintain super conductors there needs to be an extremely cold environment, which is made by using liquid helium which is only 3° above absolute zero (−452°F or −270°C). This is all housed within a large machine that is above the subjects head, which can be seen in the Figures below.
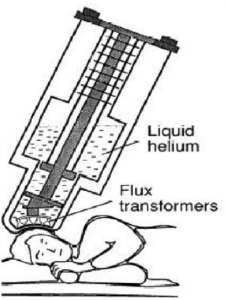
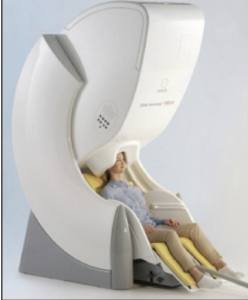
Due to the fact that the magnetic fields of interest are so small, special rooms that are shielded from magnetic fields in the environment are needed in order to avoid contamination of the signal being measured. The actual sensors recording magnetic fields are magnetometers and/or gradiometers. There are 2 types of gradiometers — axial and planar. Magnetometers provide the best signal and are most sensitive to deep brain sources but are also more sensitive to competing magnetic noise. Gradiometers are better at noise reduction. Using these sensors, MEG fields pass through the head without any distortion and provides a high spatial and temporal resolution.
MEG records magnetic fields generated by electric currents in the brain. An electric current is always associated with a magnetic field perpendicular to its direction as per the right-hand rule. The magnetic permeability of biological tissues is almost the same as that of empty space and so the magnetic field is not distorted by scalp or skull. However, the magnetic fields diminish as 1/r3 with the distance of ‘r’.
The usual amplitude of magnetic fields created by the brain are extremely small, they do not exceed a few hundred femto tesla (10-15 T). Compared with this the Earth’s magnetic field is between 10-4 T and 10-5 T and an MRI is usually between 1.5 to 3 T.
MEG currently has two approved indications in the United States, one is for pre-operative Brain Mapping and the other is for use in epilepsy surgery. MEG studies have also shown functional brain tissue inside brain tumors.
Strengths and Limitations
Learning Objectives
Type your learning objectives here.
- Explain why MEG has good temporal resolution.
- Explain what signals MEG can’t detect and how its spatial resolution compares to EEG
MEG does not detect radial dipoles but tangential dipoles are seen on MEG, this is because the corresponding magnetic field of the radial dipole remains within the cranial cavity and thus cannot be detected by sensors on the outside.
The MEG has a basic source identification problem. Determining the active site in the brain from magnetic fields recorded outside the head presents the “inverse problem”. This problem has no unique solution. Thus, source localization represents approximations based on certain assumptions that have to be made. A commonly used assumption is presuming simplified models of neuronal generators like equivalent current dipole.
MEG fields pass through the head without any distortion, which is a significant advantage of MEG over EEG. MEG provides a high spatial and temporal resolution and is more sensitive in detecting currents that are tangential to the surface of the scalp compared to EEG. MEG requires 3-4 cm2 of synchronized cortical epileptic activity to detect an epileptic spike, whereas at least 6-20 cm2 of synchronized cortical area is needed for scalp EEG spike detection. Magnetic fields are not distorted by the tissue conductivity of the scalp, skull, cerebrospinal fluid (CSF) and brain; in contrast, electrical fields may be distorted by the skull and CSF. MEG provides better spatial resolution of source localization (2-3 mm) than EEG (7-10 mm).
MEG is not as susceptible to distortions from the skull and scalp. Magnetic fields are able to pass through the hard and soft tissue relatively unchanged, which then provides better spatial resolution than EEG. MEG analytic strategies are nearly identical to those used in EEG. However, the MEG recording apparatus is more expensive than EEG, so MEG is less widely available.
Section 1. “Psychophysiological Methods in Neuroscience” by NOBA. Licensed CC-BY-NC-SA 4.0, “Magnetoencephalography: Basic principles” by NIH. Licensed CC-BY-NC-SA 3.0
Section 2. “Magnetoencephalography: Basic principles” by NIH. Licensed CC-BY-NC-SA 3.0,“Magnetoencephalography: an emerging neuroimaging tool for studying normal and abnormal human brain development” by DOAB. Licensed CC BY 4.0