Neuroscience
Nervous systems
Learning Objectives
- Know how we categorize different nervous systems in the body
- Be able to name one thing that is different between the central and peripheral nervous systems
The nervous system can be divided into four major regions: the central nervous system (CNS) and the peripheral nervous system (PNS), the sympathetic and parasympathetic nervous system, and the enteric nervous system. We’ll focus here on the CNS and PNS.
The central nervous system consists of the brain and spinal cord; the peripheral nervous system consists of everything else. The spinal cord transmits information for the brain to process. The brain is contained within the cranial cavity of the skull, and the spinal cord is contained within the vertebral cavity of the vertebral column.
The peripheral nervous system is like a system that collects information and sends commands. The PNS is so named because it is on the periphery—meaning beyond the brain and spinal cord.
Neurons in the central nervous system cannot regenerate or grow back after injury. The most obvious example of this is paralysis after spinal cord injury. Peripheral neurons, on the other hand, often do regrow after injury. For example, after a deep cut on the skin, the area around the cut is often numb for a long time after the cut looks like it has healed. But slowly—after several weeks or months—the sensation comes back.
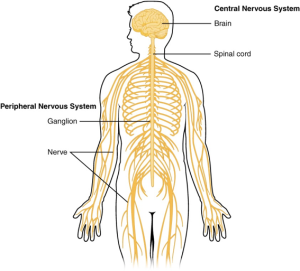
Brain Anatomy
Learning Objectives
- Know the major sub-divisions of the brain (cortex, cerebellum, thalamus)
- Know the major terms to talk about directions in the brain (neuroscience terms for up/down, front/back)
T
Neurons
Learning Objectives
- Be able to name the separate functions of axons, dendrites, and cell bodies
- Be able to describe membrane potentials and action potentials to a neighbor
Neurons are the largest population of cells in the nervous system that specialize in communication. They can come in several shapes and sizes depending on their specialized functions but all neurons will have axons and dendrites that protrude from the cell body.
There are almost as many glial cells as there are neurons in the body’s nervous system. Glial cells mostly act as caretaker cells, supporting the neurons and their connections. There are several different types.
Parts of a neuron
Dendrites are specialized structures for receiving and integrating signals from other cells. They do this mainly through receptors for small molecules and gases that are released from specialized structures at the end of the axons of other neurons.
Most neurons have a single axon that typically sends electrical impulses outwards away from the cell body. Axons can have branches, or collaterals, that can connect with other cells, or groups of cells. Axons in the PNS often travel in bundles known as nerves.
Synapses
Neurons communicate through axon-dendrite, axon-soma and sometimes dendrite-dendrite connections but these protrusions don’t actually touch. A chemical synapse consists of a small gap between two neurons, with specialized proteins at both the presynaptic and postsynaptic membrane. Processes activated by these electrical signals at the presynaptic membrane of the end of one axon result in a downstream cascade leading to the release of chemicals called neurotransmitters. Electrical synapses also exist between cells that are very close to each other and contain specialized tunnel-like structures, called gap junctions, allowing electrical signals to travel through them.
An electrical signal from one neuron’s axon will trigger a release of neurotransmitters which bind to channels on another neuron’s dendrite. An excitatory signal will either directly open up ion channels that let positively-charged ions in or trigger a series of molecular actions inside the cell that raise the membrane potential. If this increases the charge enough, it can trigger an action potential, causing that neuron to send an electrical signal (positive charge) down its own axon.
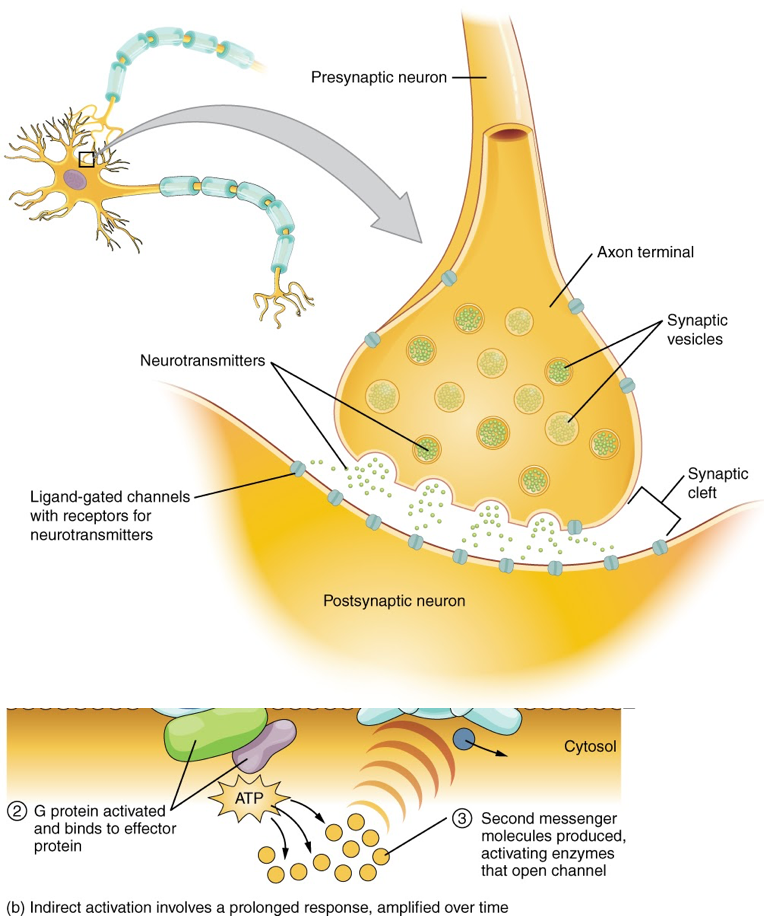
Neurotransmitters
Neurotransmitters are the biochemical messengers, or couriers, of information between cells, released from neurons at the presynaptic nerve terminal to cross through synapses where they may be accepted by a receptor site on the other side. A single neuron will produce several different neurotransmitters. The effect of a neurotransmitter is dependent on the receptor that it binds; depending on the receptor’s machinery, it may result in changes affecting the excitability of the cell or even more subtle chemical and molecular changes.
Receptors are protein structures on the surface or inside of cells that recognize and bind to specific neurotransmitters, hormones, or psychotropic drugs. Once bound, the receptor then changes shape to exert a specific effect – this may involve altering the charge of the cell by opening or closing ion channels, or more subtle effects by impacting which genes in the cell are activated. This impacts the function of the neuron; it is important to note that the effect of a neurotransmitter is entirely dependent on the receptor to which it is bound.
Action potentials and membrane potential
The functions of the nervous system—sensation, integration, and response—depend on the functions of the neurons underlying these pathways. To understand how neurons are able to communicate, it is necessary to describe the role of an excitable membrane in generating these signals. The basis of this communication is the action potential, which demonstrates how changes in the membrane can constitute a signal.
Most cells in the body make use of ions to build up a charge across the cell membrane. The cell membrane is primarily responsible for regulating what can cross the membrane and what stays on only one side. The cell membrane is a phospholipid bilayer, so only substances that can pass directly through the hydrophobic core can diffuse through unaided. Charged particles, which are hydrophilic by definition, cannot pass through the cell membrane without assistance. Channel proteins make the passage of charged particles through the membrane possible. Several passive transport channels and active transport pumps are necessary to generate a transmembrane potential and an action potential.
The carrier protein referred to as the sodium/potassium pump that moves sodium ions (Na+) out of a cell and potassium ions (K+) into a cell, thus regulating ion concentration on both sides of the cell membrane is very important when studying the brain.
A voltage-gated channel is a channel that responds to changes in the electrical properties of the membrane in which it is embedded. Normally, the inner portion of the membrane is at a negative voltage. When that voltage becomes less negative, the channel begins to allow ions to cross the membrane.
The electrical state of the cell membrane can have several variations. These are all variations in the membrane potential. A potential is a distribution of charge across the cell membrane, measured in millivolts (mV). The standard is to compare the inside of the cell relative to the outside, so the membrane potential is a value representing the charge on the intracellular side of the membrane based on the outside being zero, relatively speaking. The concentration of ions in extracellular and intracellular fluids is largely balanced, with a net neutral charge. However, a slight difference in charge occurs right at the membrane surface, both internally and externally. It is the difference in this very limited region that has all the power in neurons (and muscle cells) to generate electrical signals, including action potentials.
When the cell is at rest, the ion channels are closed and ions are distributed across the membrane in a very predictable way. Resting membrane potential describes the steady state of the cell, which is a dynamic process that is balanced by ion leakage and ion pumping. Without any outside influence, it will not change. To get an electrical signal started, the membrane potential has to change. The concentration gradient for Na+ is so strong that it will continue to enter the cell even after the membrane potential has become zero, so that the voltage immediately around the pore begins to become positive. The electrical gradient also plays a role.
Depolarization is when the membrane voltage approaches zero from the resting potential around -70mV because Na+ ions rush into the cell. Repolarization occurs as the cell tries to reset its voltage by allowing K+ out of the cell, causing the membrane voltage to return to around -70mV.
Because of the threshold, the action potential either happens or it does not. If the threshold is not reached, then no action potential occurs. However, a stronger stimulus, which might depolarize the membrane well past the threshold, will not make a “bigger” action potential. While an action potential is in progress, another one cannot be initiated. That effect is referred to as the refractory period.
The action potential is initiated at the beginning of the axon, at what is called the initial segment. There is a high density of voltage-gated Na+ channels so that rapid depolarization can take place here. Going down the length of the axon, the action potential is propagated because more voltage-gated Na+ channels are opened as the depolarization spreads.
The neuron transmitting the signal is called the presynaptic neuron, and the neuron receiving the signal is called the postsynaptic neuron. Note that these designations are relative to a particular synapse—most neurons are both presynaptic and postsynaptic. The effect of a neurotransmitter on the postsynaptic element is entirely dependent on the receptor protein. If there is no receptor protein in the membrane of the postsynaptic element, then the neurotransmitter has no effect. The depolarizing or hyperpolarizing effect is also dependent on the receptor.
Brain Anatomy: “The Central and Peripheral Nervous System” by Students of PSY 3031 and Dr. Cheryl Olman at the University of Minnesota. Licensed (?)
Neurons: “Action Potential”
Exercises