fMRI – functional MRI
Physical and Physiological Basis
Learning Objectives
- Be able to describe what signals fMRI detects
- Be able to summarize the technology involved
Although the origins of who discovered functional magnetic resonance imaging (fMRI) is debated, this neuroimaging technique is vital for the future of neuroscience. When the technology was first developed, it was sometimes called Nuclear Magnetic Resonance Imaging (NMRI) because the nuclei of the atoms are what resonates with the magnetic field. However, the word “nuclear” suggested radiation and gave people the wrong idea. Since then, they changed the name to MRI to convey that it is a relatively low-energy and safe technique (even more safe than X-ray).
MRI is the technology, but fMRI refers to when MRI is used on the brain. The difference is that MRI takes images and shares an anatomical snapshot of the body, whereas an fMRI shows a series of images to portray the changes in blood flow within the brain over time. “Functional,” therefore, refers to how the blood is moving in the brain, which allows us to infer the functions the brain is carrying out. Colored overlays are often used to demonstrate this change in blood flow.
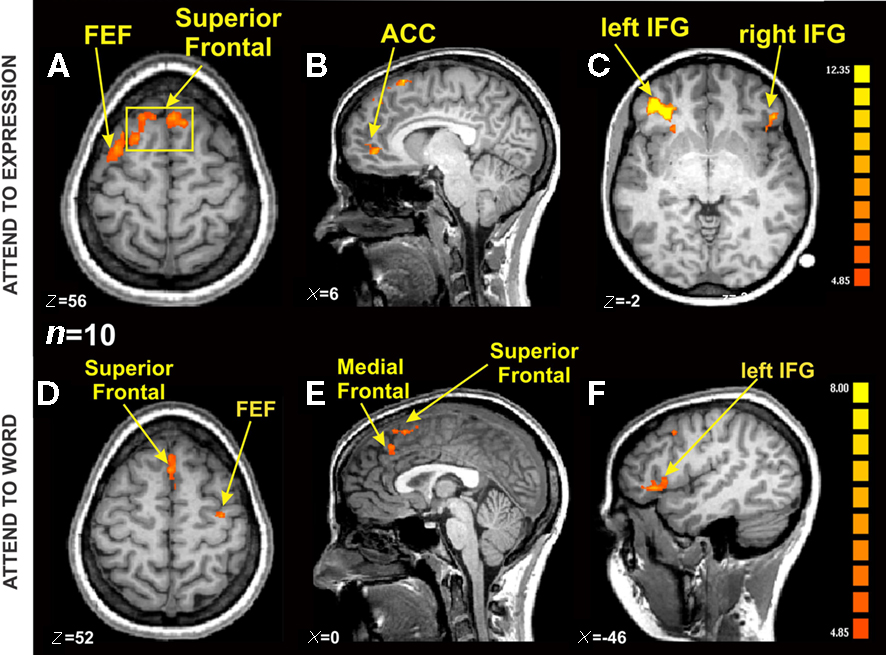
Magnetic resonance is a complicated topic, but refers to how certain frequencies of waves can align with or “resonate” with an object. Just like tuning your car radio, the antenna has to pick up a certain frequency before you will be able to hear your favorite station through the speakers. In this case, the electromagnetic field passing through your brain has to be the right frequency before the deoxyhemoglobin will react to the field and cause the imaging apparatus to pick up changes. Hemoglobin, the protein in your blood that carries and transports oxygen, contains a single Iron atom in the center that reacts differently to magnetic fields when it is carrying (oxyhemoglobin) or not carrying (deoxyhemoglobin) oxygen. When oxygen is being carried, it forms a bond with the molecule, but when it is not, the hemoglobin has unpaired iron electrons that can be more susceptible to changes from a magnetic field. When a neuron fires, it requires a rush of oxyhemoglobin to that area to replenish the oxygen needed for it to fire again. Detecting changes in blood oxygenation is involved in blood oxygenation level dependent (BOLD) signals, which is a common method used in fMRI to collect data related to activation and resting states of neurons depending on the oxygenation of that area. However, BOLD responses take time to pick up, which will be addressed in the next section.
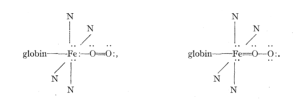
Strengths and Limitations
Learning Objectives
- Be able to explain why fMRI has poor temporal resolution
- Be able to explain what limits the spatial resolution of fMRI
While fMRI is known for its spatial resolution being better than EEG and other neuroimaging techniques, it falls short when it comes to temporal resolution. Because it takes time for the body to respond to neurons firing, it causes there to be a slight delay (4-6 seconds) before the blood oxygenation signals are picked up. This can be mitigated with more expensive scanners and software, but it is still something to note.
Functional magnetic resonance imaging is a common method of neuroimaging when high spatial resolution is needed, but it still has some difficulties. Because MRI is an anatomical-based technology, it is very good at picking up specific areas of changes. However, it can be difficult to analyze the impacts of small veins because large veins with more blood will disrupt the BOLD signals in that area or vein pooling can occur, which causes nearby veins to seem to emit the same signal (figure 3). Additionally, water molecules diffuse differently depending on the size of the vein. Therefore, there are different techniques available for evaluating more specific spatial changes, including Gradient and Spin Echo EPI (GRASE) and Vascular Space Occupancy (VASO).
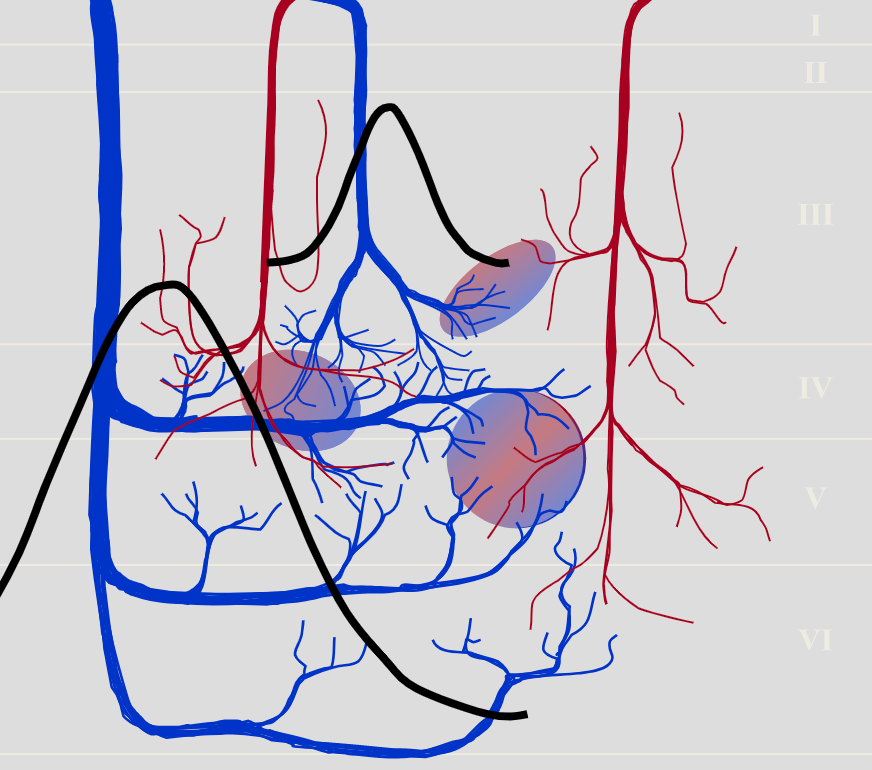
Overall, fMRI is a great technique that is used in a wide range of experiments and medical procedures. Its spatial resolution is great compared to other techniques and even its shortfalls can be addressed through using different techniques for analysis. fMRI is best used in tandem with EEG or other neuroimaging techniques with a higher temporal resolution when that component is needed, but again, there are techniques that alleviate that problem as well.
“Functional MRI: Basic Principles” by caolman. Licensed CC BY 4.0.