Climate Change: The Carbon Cycle
Carbon, just like all other elements, cycles through the environment and is constantly in the process of changing forms and locations. In this section, as in many other pieces of scientific literature, we will periodically refer to carbon by its chemical symbol, C. There is no new carbon in the world, rather, all carbon is continuously recycled from one form to another. All plants, animals (including humans!), fungi, bacteria, and archaea are made of mostly carbon-based molecules such as lipids, carbohydrates, proteins, and nucleic acids. Carbon is also prevalent in soils, rocks and sediments, water bodies (dissolved), and the atmosphere. These locations where carbon resides are known as pools or reservoirs, and the processes that move carbon from one location to another are called fluxes. Figure 7.1 shows a simplified version of the global carbon cycle.
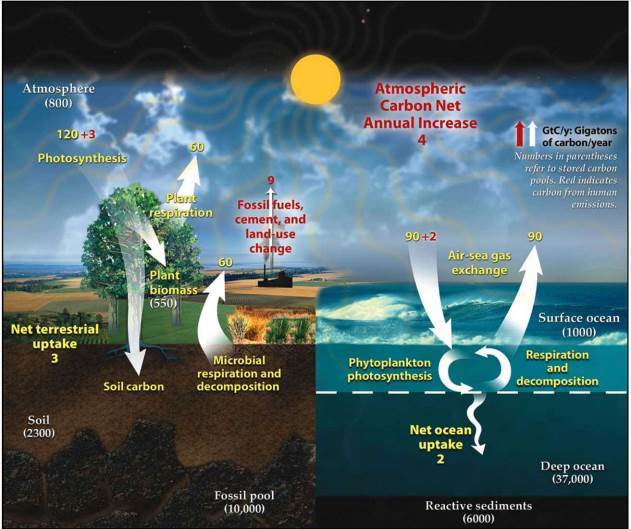
Some reservoirs hold onto carbon for only a short time. Aerobic (oxygen-using) organisms convert carbohydrates created by other organisms into carbon dioxide (CO2) almost instantaneously, which they exhale into the atmosphere. When considering the flux of respiration, living organisms are the source of carbon, and the atmosphere is the sink. The carbon stays in the reservoir of living organisms for a relatively short time, depending on their life span, from hours and days to years and decades. In contrast, the residence time of carbon in the fossil pool is dramatically different. Fossil fuels form over 300-400 million years from ancient plants and animals that decomposed slowly under very specific, anaerobic (without oxygen) conditions in wetland environments. Their bodies were gradually transformed by the heat and pressure of the Earth’s crust into the fossil fuels that we mine today to provide petroleum oil, natural gas, and coal (see more on this in chapter 4).
Important Reservoirs
The two largest reservoirs of carbon on Earth are the oceans, which cover the majority of Earth’s surface, and the lithosphere (the mineral fraction of Earth: soils, rocks, and sediments). Each of these reservoirs holds more carbon than all of the other reservoirs combined. Much of the carbon stored in these reservoirs, especially deep in the lithosphere or in deep ocean environments, has an extremely long residence time and does not actively participate in rapid fluxes. The notable exceptions here are fossil fuels, which are mined by humans and converted into gaseous forms of carbon through combustion. Biomass, which is biological material derived from living, or recently living organisms, is a much smaller reservoir of carbon. The amount of carbon stored in all of the terrestrial vegetation (550 Gt C) (Gt = gigatonne = 109 metric tons = 1015 g) is just a fraction of that stored in the oceans (38,000 Gt C) and lithosphere (18,000 Gt C).
Important Fluxes
Photosynthesis
All of the carbon that is stored in Earth’s vegetation got there through the process of photosynthesis. Plants and other photosynthetic organisms are called primary producers, because they “fix” atmospheric CO2 into organic carbon, such as sugar, a form that is usable by animals and other organisms that need to consume their carbon molecules.
Photosynthetic organisms, such as plants, algae, and cyanobacteria, bring in CO2 from the atmosphere and use the energy from the sun to convert CO2 and water into glucose molecules (organic carbon). The products of photosynthesis are oxygen and glucose (Equation 7.1). These glucose molecules are simple sugars that autotrophs (“self-feeders”) can “burn” for energy, or transform into other usable carbon molecules through the process of cellular respiration (see next paragraph), or to build plant biomass. Photosynthesis takes place in organelles called chloroplasts, shown in Figure 7.2. Photosynthesis accounts for 123 Gt of C per year which is removed from the atmosphere and stored in plant biomass. Such a massive amount of photosynthesis occurs on Earth that no other single flux moves as much carbon in the same timeframe.
Eq. 7.1
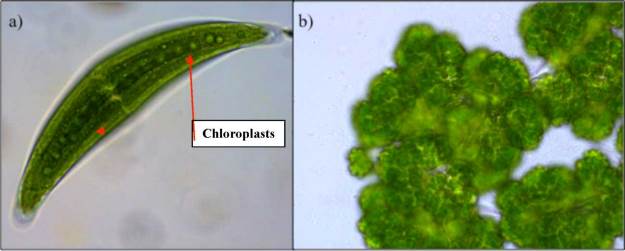
Cellular Respiration
Biomass, including plants and animals, is the reservoir of carbon that we are most likely most familiar with and the reservoir that is most readily available to us. We all participate in the flux of consumption of carbon when we eat food. All of our food is simply plant and/or animal biomass. Our body takes the carbon molecules contained in this biomass, and uses them, along with the oxygen we breathe in, for cellular respiration to create the adenosine triphosphate (ATP) we need for energy. The products of cellular respiration include the CO2 we exhale, water, and energy that is stored in ATP (Equation 7.2). Our bodies also build additional biomass out of the carbon molecules in this food, allowing us to create new cells for growth or replenishment. This is the only way we, and all other heterotrophs (“other-eaters”), can bring in the carbon we need to build and maintain our bodies. Remember, you are what you eat!
Eq. 7.2
Cellular respiration is an important flux in the carbon cycle and one that contributes carbon to the atmosphere. Remember that animals and other heterotrophs complete cellular respiration using the carbon molecules they bring in through their food. Plants and other photosynthetic autotrophs complete cellular respiration using the carbon molecules they formed from CO2 through photosynthesis. Any carbon molecules that are left over after the organism has acquired sufficient energy through cellular respiration make up the biomass of the plant.
Decomposition
As plants and animals die and decompose, their bodies are consumed by decomposers organisms such as fungi and bacteria. Through the flux of decomposition, some decaying biomass is converted into atmospheric carbon by the decomposers, while most of the biomass is buried in the soil, contributing to soil carbon. In oxygen-rich environments, decomposers rapidly consume dead and decaying biomass using the same process of aerobic cellular respiration described above. In oxygen-deficient environments, decomposers complete other metabolic pathways and very slowly consume the organic matter. Some of the gases produced from anaerobic decomposition include methane (CH4), nitrous oxide (N2O), and the foul-smelling hydrogen sulfide (H2S).
Combustion
The biomass reservoir of the carbon cycle is also important to us as a source of energy. Through the flux of combustion, we convert the potential energy held in biomass into heat energy that we can use, and release carbon dioxide in the process. If you have ever burned logs on a campfire or even burned food on the stove, you have completed this flux of biomass combustion. Of course, this happens naturally as well, the best example being natural forest fires caused by lightning strikes. The chemical reaction for combustion is identical to the chemical reaction for cellular respiration. The difference is that in cellular respiration, energy is released in a controlled fashion, and captured in ATP molecules. In combustion, all of this energy is released rapidly in the form of light and heat.
Ocean-Atmosphere Exchange
As all of the fluxes we’ve discussed so far involve the atmosphere, we have not yet discussed the flux that connects the atmosphere to the oceans. Carbon can enter the oceans through two primary fluxes: first through photosynthesis by algae or cyanobacteria (also called phytoplankton in Figure 7.1), and second through the chemical reaction of ocean-atmosphere exchange. The ocean, as with all surface water bodies, always contains some dissolved CO2. This CO2 is in equilibrium with the CO2 in the air. Some atmospheric CO2 is constantly dissolving into the ocean, while some dissolved CO2 is constantly diffusing into the atmosphere. Under normal conditions, these two fluxes will be happening at equal rates. As you can see in Figure 7.1, however, this is no longer the case. In the section Human impacts on the carbon cycle, we will discuss why this is the case.
Activity: Better understanding of the carbon cycle
To further review the carbon cycle, and better understand the human impacts on it, use this interactive graphic from Woods Hole laboratories: http://www.whoi.edu/feature/carboncycle/. As you will see, the information described in this text is only a small portion of the total carbon cycle on Earth. Finally, complete Table 7.1 as a way to review the sink/source relationship within this cycle. See if you can correctly identify the source and sink of carbon for each of these important fluxes in the carbon cycle.
Table 7.1. Practice understanding the sink/source relationship with cycles
Carbon flux |
Carbon Source |
Carbon sink |
Cellular respiration |
Carbohydrates in living organisms |
CO, in the atmosphere |
Photosynthesis |
|
|
Consumption |
|
|
Combustion |
|
|
Decomposition |
|
|
Ocean/atmosphere exchange |
|
|
Fossil fuel formation |
|
|
Human impacts on the carbon cycle
Humans, just like all other living organisms, have impacted the global carbon cycle since the dawn of our species. However, the magnitude of our impacts has changed dramatically throughout history. The Industrial Revolution, which occurred around the turn of the 19th century, began to make major changes in the use of resources around the world. Beginning in Britain, industrialization eventually affected the whole world. The development of coal-fueled steam power, and later transportation following the discovery of large oil deposits, had enormous influence on the economic and social structure of the world. As the world accelerated in the production and transportation of manufactured goods, the production and consumption of fossil fuels grew. As economic growth continued to increase, so did the production of carbon dioxide through fossil fuel combustion. See Figure 7.4 later in this text.
Some of the human impacts on the carbon cycle have been quantified for you in Figure 7.1. Changes to fluxes in the carbon cycle that humans are responsible for include increased contribution of CO2 and other greenhouse gases to the atmosphere through the combustion of fossil fuels and biomass; increased contribution of CO2 to the atmosphere due to land-use changes; increased CO2 dissolving into the ocean through ocean-atmosphere exchange; and increased terrestrial photosynthesis. The first two impacts, both contributing excess CO2 to the atmosphere at a rate of 4 Gt of carbon per year have, by far, the largest impact on our planet. For this reason, this is the change that we will most often focus on throughout this section. The excess CO2 in the atmosphere is responsible for the increased CO2 dissolving into the ocean, which we will discuss later in this section. This is also, in part, responsible for the increased terrestrial photosynthesis that can be observed, as additional CO2 is available to plants for photosynthesis. However, intensive agricultural and forestry practices also contribute to the change in this flux.
One characteristic example of a human impact on the carbon cycle is illustrated in Figure 7.3. Throughout most of our recent human history, people have been physically altering the landscape around them to have more control over their surroundings and increase their odds of survival. One way that people have done this is through agriculture. For most forms of agriculture to be successful, native vegetation is eliminated or minimized. Resources from this native vegetation, such as wood, may be used for combustion to provide heat, sanitation, or fuel for cooking. Combustion may also be used as an efficient way to clear the land and make way for crops or grazing lands for livestock. Often, settlements are formed around these newly fashioned agricultural fields, and the land is used similarly for many years in the future.
Let’s identify how humans are impacting the carbon cycle in this scenario of agricultural establishment. You should be able to identify from the above paragraph that the flux of combustion will release CO2 previously held in vegetation into the atmosphere. In addition, remember that the land that used to house native vegetation is now home to agricultural lands. In most controlled agricultural environments, there is less total vegetative biomass than there would be under natural conditions. This decreased biomass leads to lower total photosynthesis rates, thereby decreasing the amount of CO2 that is removed from the atmosphere and turned into plant biomass. Also, open soil on the fields between crops, during the winter months, or as a result of overgrazing allows for the air to penetrate deep into the soil structure. This provides the environment necessary for enhanced aerobic respiration by soil microorganisms. This decreases soil carbon, which can lead to erosion and soil degradation, and also releases additional CO2 into the atmosphere.
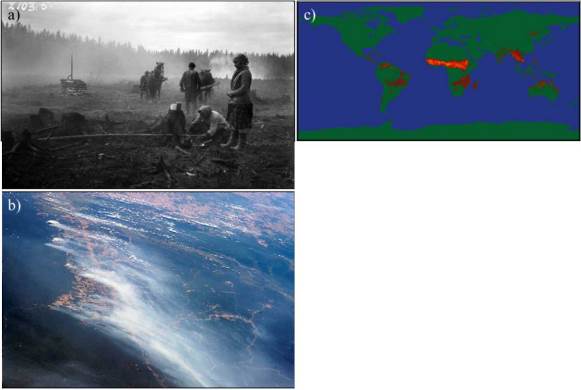
As you learned in Chapter 5, biomass is an important form of energy for human civilization. Before the Industrial Revolution, this was essentially the only form of fuel to which most people on Earth had access. In many less-industrialized countries, the combustion of biomass such as wood or animal dung is still the primary energy source that many citizens, particularly in rural areas, depend on for domestic use (heating, sanitation, and cooking) as it is inexpensive, relatively efficient, and readily available. Figure 7.3c shows the global distribution of biomass fires in the world. While the burning of biomass for domestic use contributes to some of these fires, it is the so-called slash-and-burn agriculture that makes up a larger contribution. Take a minute to compare the areas highlighted in Figure 7.3c to the countries of the world that are currently experiencing rapid population growth (Chapter 3). If you need a refresher, use the CIA World Factbook website to view current global population growth values by country: https://www.cia.gov/library/publications/the-world-factbook/rankorder/2002rank.html.
While biomass burning still has a significant impact on the global carbon cycle, human impacts on fluxes such as fossil fuel extraction and combustion continue to grow. For a review of the impacts of non-renewable energy sources such as fossil fuels, see Chapter 4. Burning of any fossil fuel (coal, natural gas, crude oil) moves carbon from a previously-sequestered state deep within the Earth’s crust into carbon dioxide in the atmosphere. As countries become more industrialized, their reliance on and combustion of fossil fuels tends to increase. Look at the graph in Figure 7.4, which compares CO2 emissions from fossil fuels of regions across the globe.
How has the use and distribution of fossil fuels changed throughout the past 250 years?
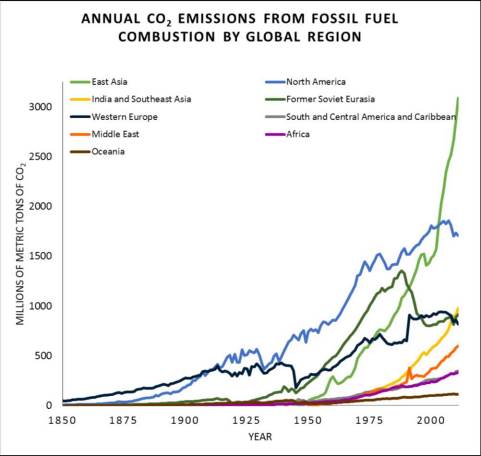
The data shown in Figure 7.4 reveals much about the regions of the world it depicts. The effects of historical events such as the Great Depression of 1929-1939, World Wars, the fall of the Soviet Union in 1991, and the Kuwait oil fires of 1991 can be seen. Furthermore, between 1850 and 2011, different regions have gone in and out of the lead position as top producers of CO2 from fossil fuel emissions. Population is one reason why fossil fuel use has changed throughout time. This is particularly apparent when comparing the data for Western Europe to that of India and Southeast Asia.
As countries industrialize, their relationship with agriculture also changes. More industrialized countries rely very little on slash-and-burn agriculture. Their agricultural practices, however, are no less impactful on the environment. The growing population (Chapter 3) in many countries has required agriculture to become industrialized to meet demand. As a person living in the United States, industrialized agriculture probably produces the vast majority of the food you eat, including grains, fruits and vegetables, dairy and eggs, meats, and even fish. Industrialized agriculture can refer to a variety of practices, but has several main components: the use of motorized machinery; the use of chemicals such as fertilizers, pesticides, hormones, and/or antibiotics; and the intense and efficient production of one product across a large area of land.
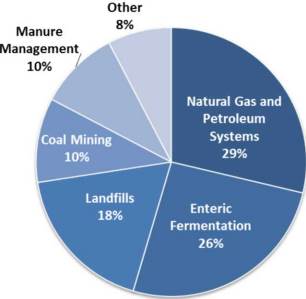
One example of the impacts of industrialized agriculture is the production of methane (CH4), a potent greenhouse gas. You will learn more about methane later in this section. As you saw earlier, methane is a common product of anaerobic metabolisms. The gut of ruminant animals (such as sheep, cattle, and goats) has evolved to allow the animals to digest the very tough carbon molecules, such as cellulose, in the grass. They do this through symbiosis, or cooperation, with anaerobic bacteria that live in the gut tract. These anaerobic bacteria produce methane and other gases as a result of their metabolism when they break down molecules like cellulose. This is sometimes called enteric fermentation. The methane gas is excreted from the animal, and this contributes significantly to total methane emissions (Figure 7.5). A similar type of bacteria live in the fecal matter, or manure, of livestock. As the manure is handled or stored for future use, methane is also released into the environment.
The methane excretions of one cow or a few sheep would be minuscule and insignificant. If you were a small farmer with only enough livestock to feed your family, your contribution to total methane emissions would be close to zero. However, the demand for animal protein from meat, dairy, and eggs is very large in the United States. As of January 2015, the United States had a total cattle inventory of 89.9 million animals, and in 2014, 25.5 billion pounds of beef was consumed in the United States (statistics: National Cattlemen’s Beef Association). The impacts of enteric fermentation and manure management for almost 90 million animals are very significant, as seen in Figure 7.5. In both cases, carbon that was previously stored in biomass (cattle feed) is moved into the atmosphere, this time in the form of CH4. This is another example of how humans have impacted the carbon cycle.
Previously in this chapter, you identified other ways the carbon cycle is impacted by human agriculture. Through industrialized agriculture, we must also account for the fossil fuels that are used. To deliver agricultural products to consumers, fossil fuels are used numerous times: deliveries of fertilizer, feed, and/or seed to farms; farm machinery; delivery of products to processers; food processing; delivery of foods to supermarkets; etc.
As animal products, especially meat, are expensive, the demand is typically greater in more industrialized countries than it is in less industrialized countries. This makes industrialized agriculture, and especially industrialized animal agriculture, one of the major contributors to greenhouse gas emissions in more industrialized countries.
Knowledge check – answer these questions on your own to further explore the impacts of biomass and fossil fuel burning on the global carbon cycle.
1. Why is there a correlation between population growth rate and global distribution of biomass fires?
2. Do you think this correlation is more likely due to personal biomass fires for activities such as cooking, or due to slash-and-burn agriculture? Why?
3. Given any other knowledge you might have about the areas highlighted in Figure 7.3c, what other environmental impacts may be occurring here besides carbon cycle alterations?
4. Compare the production of CO2 emissions from fossil fuel combustion across world regions in 1900, 1950, and 2011 in Figure 7.4. What has accounted for these differences?
5. Has the total worldwide production of CO2 from fossil fuels increased evenly relative to human population growth during the period displayed in Figure 7.4? Why or why not?
6. What are the differences in contributions of greenhouse gas emissions from more-industrialized countries and less-industrialized countries? What are the similarities?
Resources
Carbon Dioxide Information Analysis Center http://cdiac.esd.ornl.gov/
NOAA Earth System Research Laboratory: Carbon Cycle Science http://www.esrl.noaa.gov/research/themes/carbon/
Sass, Ronald. Q2: What are the Causes of Global Climate Change? OpenStax CNX. Sep 22, 2009 http://cnx.org/contents/5d263a29-7bd6-47bf-ad70-c233619bca33@3
USDA Climate Change and Agriculture in the United States: Effects and Adaptation http://www.usda.gov/oce/climate_change/effects_2012/effects_agriculture.htm
US EPA Overview of Greenhouse Gases: Methane http://epa.gov/climatechange/ghgemissions/gases/ch4.html
Woods Hole Oceanographic Institution: Carbon Around the Earth http://www.whoi.edu/feature/carboncycle/
Attribution
Zehnder, Caralyn; Manoylov, Kalina; Mutiti, Samuel; Mutiti, Christine; VandeVoort, Allison; and Bennett, Donna, “Introduction to Environmental Science: 2nd Edition” (2018). Biological Sciences Open Textbooks. 4.
https://oer.galileo.usg.edu/biology-textbooks/4
This work is licensed under a Creative Commons Attribution-Noncommercial-Share Alike 4.0 License.