25 Natural Selection and Adaptation
Stabilizing Selection
If natural selection favors an average phenotype, selecting against extreme variation, the population will undergo stabilizing selection (Figure 1). In a mouse population that live in the woods, for example, natural selection is likely to favor mice that best blend in with the forest floor and are less likely for predators to spot. Assuming the ground is a fairly consistent shade of brown, those mice whose fur is most closely matched to that color will be most likely to survive and reproduce, passing on their genes for their brown coat. Mice that carry alleles that make them a bit lighter or a bit darker will stand out against the ground and be more likely to fall victim to predation. As a result of this selection, the population’s genetic variance will decrease.
Directional Selection
When the environment changes, populations will often undergo directional selection (Figure 1), which selects for phenotypes at one end of the spectrum of existing variation. A classic example of this type of selection is the evolution of the peppered moth in eighteenth- and nineteenth-century England. Prior to the Industrial Revolution, the moths were predominately light in color, which allowed them to blend in with the light-colored trees and lichens in their environment. However, as soot began spewing from factories, the trees darkened, and the light-colored moths became easier for predatory birds to spot. Over time, the frequency of the moth’s melanic form increased because they had a higher survival rate in habitats affected by air pollution because their darker coloration blended with the sooty trees. Similarly, the hypothetical mouse population may evolve to take on a different coloration if something were to cause the forest floor where they live to change color. The result of this type of selection is a shift in the population’s genetic variance toward the new, fit phenotype.
LINK TO LEARNING
In science, we sometimes believe some things are true, and then new information becomes available that changes our understanding. The peppered moth story is an example: some scientists recently have questioned the facts behind the selection toward darker moths. Read this article to learn more.
Diversifying Selection
Sometimes two or more distinct phenotypes can each have their advantages for natural selection, while the intermediate phenotypes are, on average, less fit. Scientists call this diversifying selection (Figure 1) We see this in many animal populations that have multiple male forms. Large, dominant alpha males use brute force to obtain mates, while small males can sneak in for furtive copulations with the females in an alpha male’s territory. In this case, both the alpha males and the “sneaking” males will be selected for, but medium-sized males, who can’t overtake the alpha males and are too big to sneak copulations, are selected against. Diversifying selection can also occur when environmental changes favor individuals on either end of the phenotypic spectrum. Imagine a mouse population living at the beach where there is light-colored sand interspersed with patches of tall grass. In this scenario, light-colored mice that blend in with the sand would be favored, as well as dark-colored mice that can hide in the grass. Medium-colored mice, alternatively would not blend in with either the grass or the sand, and thus predators would most likely eat them. The result of this type of selection is increased genetic variance as the population becomes more diverse.
VISUAL CONNECTION
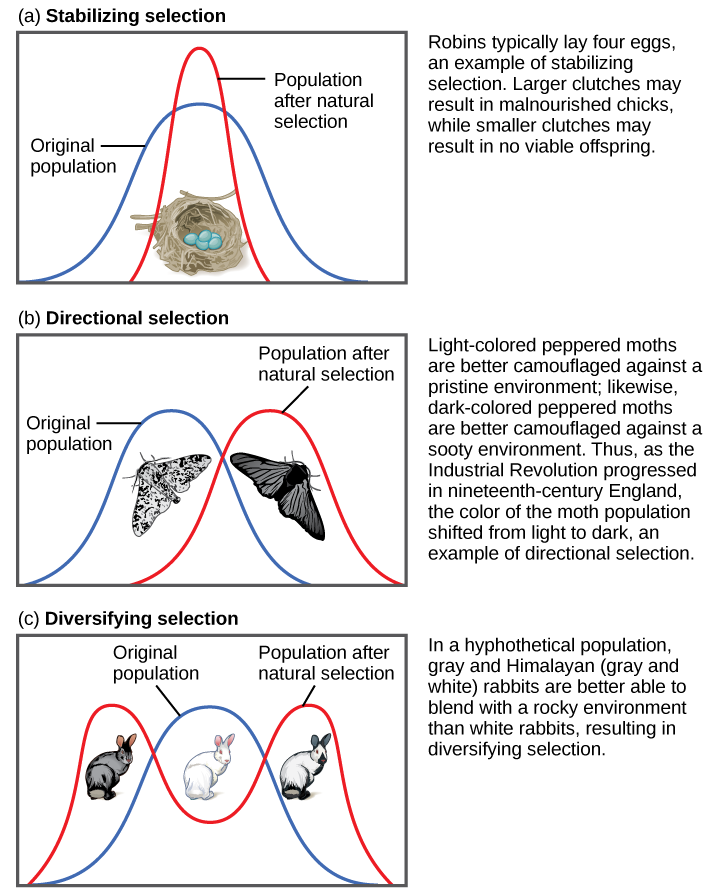
In recent years, factories have become cleaner, and release less soot into the environment. What impact do you think this has had on the distribution of moth color in the population?
Answer:
Moths have shifted to a lighter color.
No Perfect Organism
Natural selection is a driving force in evolution and can generate populations that are better adapted to survive and successfully reproduce in their environments. However, natural selection cannot produce the perfect organism. Natural selection can only select on existing variation in the population. It does not create anything from scratch. Thus, it is limited by a population’s existing genetic variance and whatever new alleles arise through mutation and gene flow.
Natural selection is also limited because it works at the individual, not allele level, and some alleles are linked due to their physical proximity in the genome, making them more likely to pass on together (linkage disequilibrium). Any given individual may carry some beneficial and some unfavorable alleles. It is the alleles’ net effect, or the organism’s fitness, upon which natural selection can act. As a result, good alleles can be lost if individuals who carry them also have several overwhelmingly bad alleles. Likewise, bad alleles can be kept if individuals who have enough good alleles to result in an overall fitness benefit carry them.
Furthermore, natural selection can be constrained by trade-offs between different traits or polymorphisms. One morph may confer a higher fitness than another, but may not increase in frequency because going from the less beneficial to the more beneficial trait would require going through a less beneficial phenotype. Think back to the mice that live at the beach. Some are light-colored and blend in with the sand, while others are dark and blend in with the patches of grass. The dark-colored mice may be, overall, more fit than the light-colored mice, and at first glance, one might expect the light-colored mice to be selected for a darker coloration. However, remember that the intermediate phenotype, a medium-colored coat, is very bad for the mice—they cannot blend in with either the sand or the grass and predators are more likely to eat them. As a result, the light-colored mice would not be selected for a dark coloration because those individuals who began moving in that direction (began selection for a darker coat) would be less fit than those that stayed light.
Finally, it is important to understand that not all evolution is adaptive. While natural selection selects the fittest individuals and often results in a more fit population overall, other forces of evolution, including genetic drift and gene flow, often do the opposite: introducing deleterious alleles to the population’s gene pool. Evolution has no purpose—it is not changing a population into a preconceived ideal. It is simply the sum of the various forces that we have described in this chapter and how they influence the population’s genetic and phenotypic variance.
Glossary
- adaptive evolution
- increase in frequency of beneficial alleles and decrease in deleterious alleles due to selection
- directional selection
- selection that favors phenotypes at one end of the spectrum of existing variation
- diversifying selection
- selection that favors two or more distinct phenotypes
- evolutionary fitness
- (also, Darwinian fitness) individual’s ability to survive and reproduce
- frequency-dependent selection
- selection that favors phenotypes that are either common (positive frequency-dependent selection) or rare (negative frequency-dependent selection)
- relative fitness
- individual’s ability to survive and reproduce relative to the rest of the population
- stabilizing selection
- selection that favors average phenotypes
Access for free at https://openstax.org/books/biology-2e/pages/1-introduction